Gut Vision is a series about the ways that we not only consume food but also symbols.
Arjun Potter is a PhD Candidate in Ecology and Evolutionary Biology at Princeton University. Arjun’s current research is on diets and grazing dynamics of 14 large herbivores and their relation to plant traits of 204 plant species in the savannas of Gorongosa national park in Mozambique. In the following conversation Arjun helps us understand the ecological and evolutionary basis of eating preferences, the meaning of nutrition and what studying herbivore diets can tell us about the future of human dietary habits. Arjun’s ecological research asks why large mammalian herbivores eat what they eat, describing their diets in great detail with DNA metabarcoding, its impact on the land and ecosystems and the very important truth: ecology is everywhere from the savannas of Africa to our own intestines.
Somnath Bhatt:
Starting on a personal note, what led you to the current research you do?
Arjun Potter:
As a child, I enjoyed birdwatching and identifying wild plants – biodiversity has inspired me for my whole life. Over time, this developed into interests in conservation and natural history. I spent one summer in high school shadowing a conservationist studying grassland birds in the Kutch district, which is in the extreme west of India. The experience was particularly memorable, as I learned about how the birds I loved so much were declining due to habitat destruction. During this trip I also found that a similar fate was impacting local pastoralists, who rely on dwindling patches of grassland to graze their cattle. Since then, I’ve been trying to find ways to protect biodiversity and to deepen the relationship between humans and nature.
After studying ecology during my undergrad at Cornell University, I spent a year in Indonesia on a Fulbright studying the impact of wild cattle on plant diversity. I developed more of an interest in the conceptual side of things, and I started a PhD in Ecology and Evolutionary Biology at Princeton University. During my PhD, I’ve continued the grassland theme, with research in the savannas of Gorongosa National Park in Mozambique. I love ecological research–it connects me to beautiful landscapes and people and some very important questions.
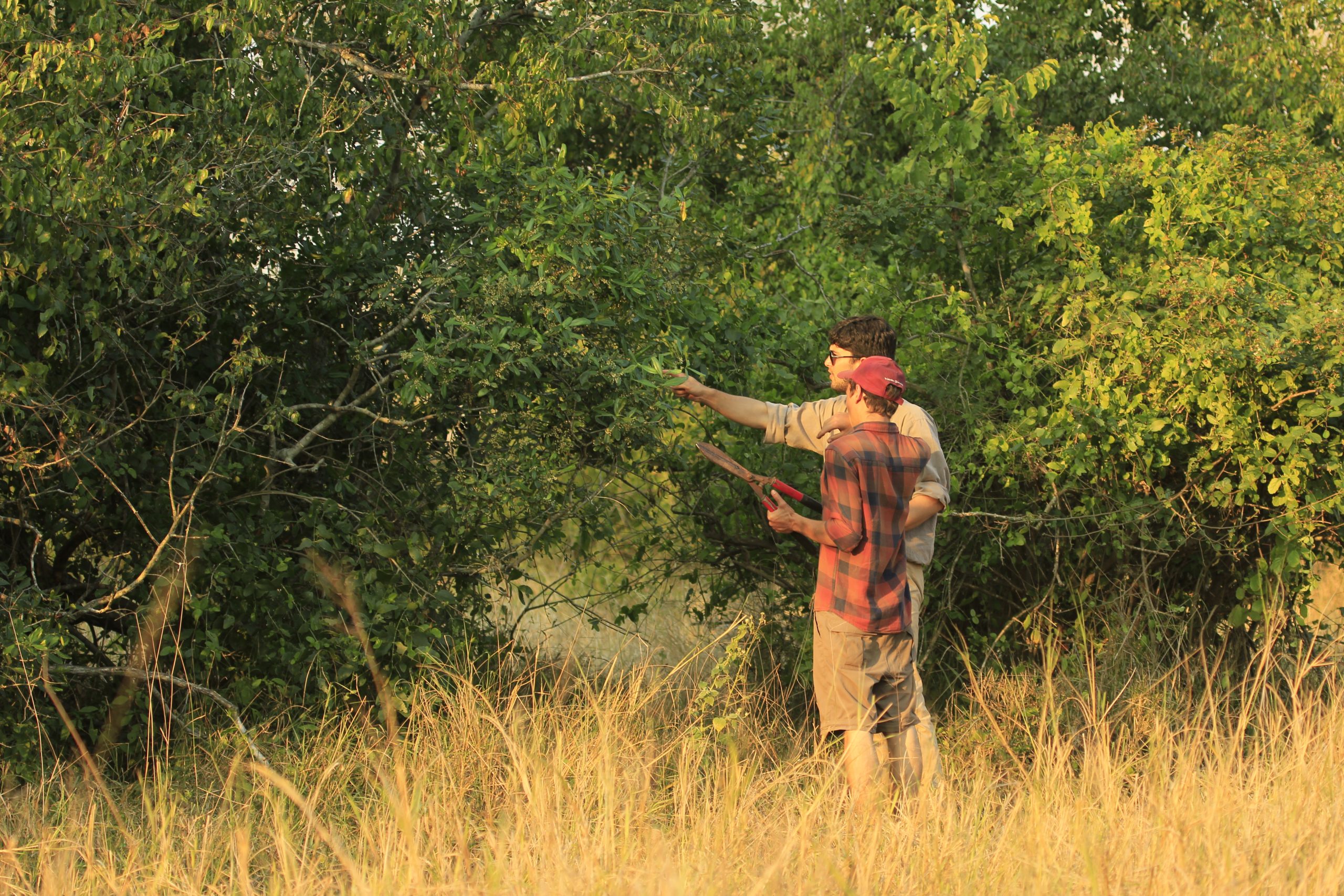
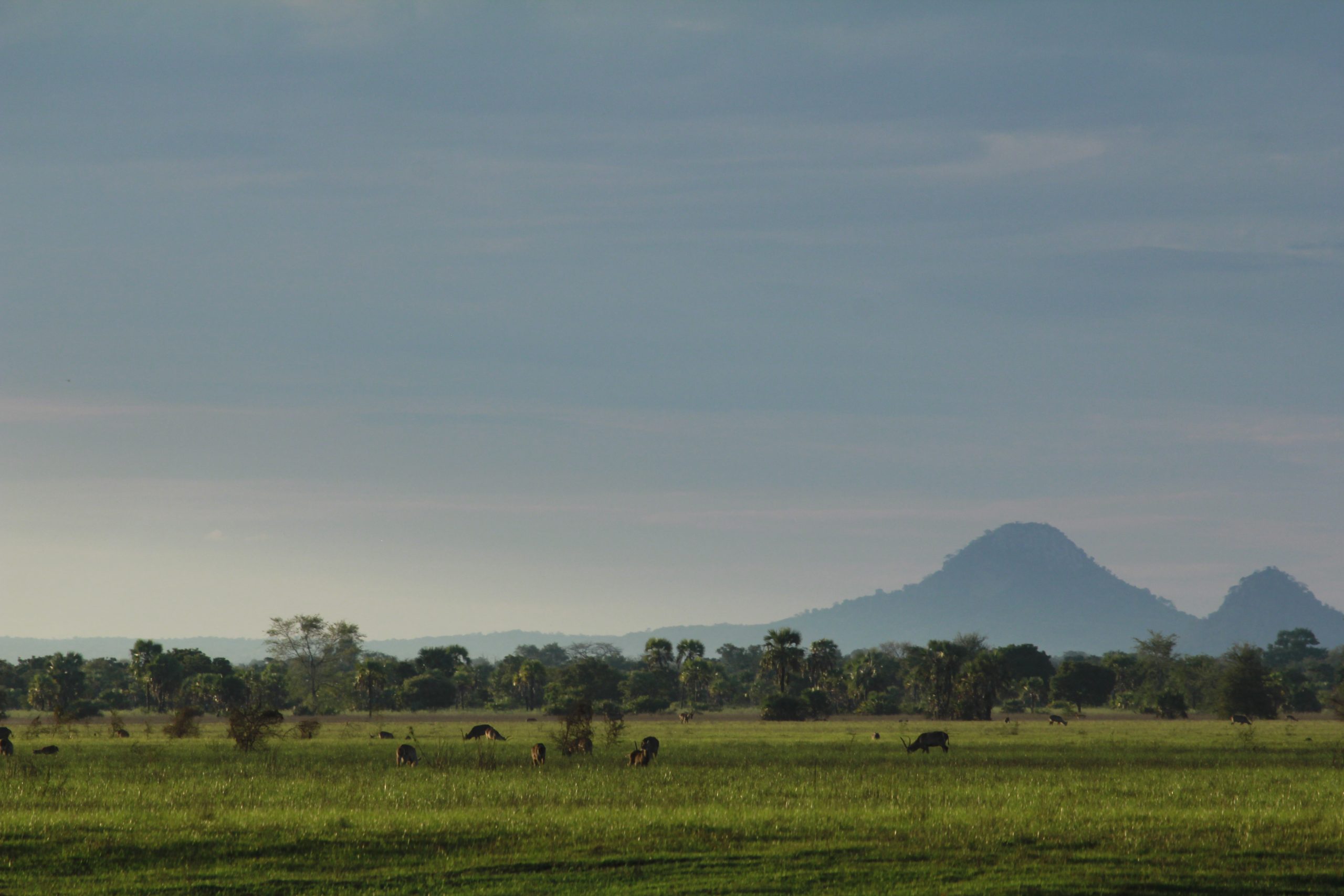
SB:
How can studying the correlation between herbivore diets and grassland ecology inform how we understand the future of human dietary habits?
AP:
To understand the future of human diets, it makes sense to look at the past. Modern humans (Homo sapiens) are generally omnivores (eating a mix of everything), but if we compare ourselves with other great apes, namely chimpanzees (Pan), gorillas (Gorilla), and orangutans (Pongo), we can infer that our ancestral diet was mostly plants. Our ancestors evolved in the forests and savannas of Africa, alongside numerous other species of primates; competition for food was a fact of life. In this way, studies of the large mammalian herbivores (even hoofed ones) give us a general understanding of the ecological conditions present for a good chunk of human evolution.
These studies suggest several things about humans:
- Humans are adapted to spend long periods of time gathering the most digestible plant material we could find (fruits, nuts, leaves)
- Humans are adapted to digest a more fibrous diet; our guts must have held a diverse community of symbiotic bacteria that would have helped us slowly break down our food
- Being somewhat large-bodied, more nimble monkeys would have beaten humans to the highest-quality food, leaving us to eat more fibrous plant matter. (Jarman-Bell principle1)
- Our diets would have been rich in plant defensive compounds, many of which are toxic in large quantities. These compounds are also what give vegetables, herbs, and spices their unique and pungent flavors. To avoid overdosing, we are likely adapted to consume these in moderation and to consume a very diverse diet to mitigate the effects of any one component. (Freeland-Janzen hypothesis)
- 1. The Jarman-Bell principle describes the tendency of large-bodied herbivores to eat lower-quality diets than small-bodied herbivores. What this means is that we would expect that a large herbivore such as a buffalo would eat a diet that is more fibrous, difficult to digest, and lower in protein and energy. In contrast, a smaller herbivore such as a goat would eat a much richer diet. The principle seems to be true; what causes this pattern is still very much up for debate!
But it is also important to remember that you don’t need to go to a national park to enjoy nature – ecology is everywhere, even in your stomach.
SB:
How would you describe the central questions of the research you are doing?
AP:
Some of the central questions of my research are:
- Why do animals eat what they eat?
Evolutionary theory would predict that animals should eat whatever maximizes their fitness—that is, animals should eat what helps them survive and reproduce. Most foods are imperfect in some way— a food might have too much of one nutrient and not enough or another, or some foods are nutritious but rare, while others are good but toxic in large quantities. Animals need to find a way to navigate all sorts of difficult choices. To figure this out, ecologists might make a prediction about what sort of food an animal would eat and then see if that is actually the case – or if there are other possibilities.
- How do so many species of animals manage to coexist in a world of limited resources? One major class of explanations for why species manage to coexist is that they occupy different niches within an ecological community.2 These differences allow species to divide up niche space and minimize competitive exclusion. But what exactly are these differences and how do they work? Using a combination of observation, experimentation, and theory, ecologists have come up with all sorts of possibilities.
- What are the implications of large body size?
Body size is incredibly important in ecology because size has all sorts of physical and physiological implications. The field of allometry, which studies the relationship between body size and its implications using a mathematical approach, seeks to describe and understand major patterns in biology.
- How do the traits of organisms influence species interactions?
One approach in ecology is to describe the traits, or characteristics, of organisms and use these to explain ecological patterns. For instance, certain plants characteristically have lots of nitrogen in their leaves (a tomato, for example); these plants seem to require lots of nitrogen in the soil. Other plants don’t need much nitrogen at all (e.g. crabgrass). We would therefore predict that without much fertilizer, grasses would do just fine and tomato plants would suffer; fertilizing would allow the fast-growing tomato plant to outstrip the grass and shade it out. In this case, the traits of grasses and tomatoes (leaf nitrogen content, relative growth rate) would determine the competitive battles inherent in vegetable gardening.
- 2. One example of different niches in an ecological community is the grazer-browser spectrum. The grazer-browser spectrum describes another mechanism for dietary niche partitioning–the concept is that large animals vary in their consumption of grass vs. browse (trees, shrubs). Some animals may eat a mix of grass and non-grass, and thus fall in the middle of the spectrum. The pattern is most evident in the African savanna, where species such as zebras, wildebeest, and warthogs eat mostly grasses, while antelopes such as kudu eat almost only tree foliage; some animal, such as African elephants for instance, seem to fall in the middle. This same pattern seems to be true in other parts of the world, including in our agricultural ecosystems–for instance, sheep are grazers and eat mostly grass, while goats are browsers and relish shrubs and weeds. For this reason, many pastoralists will herd goats and sheep together, as the two species complement each other.
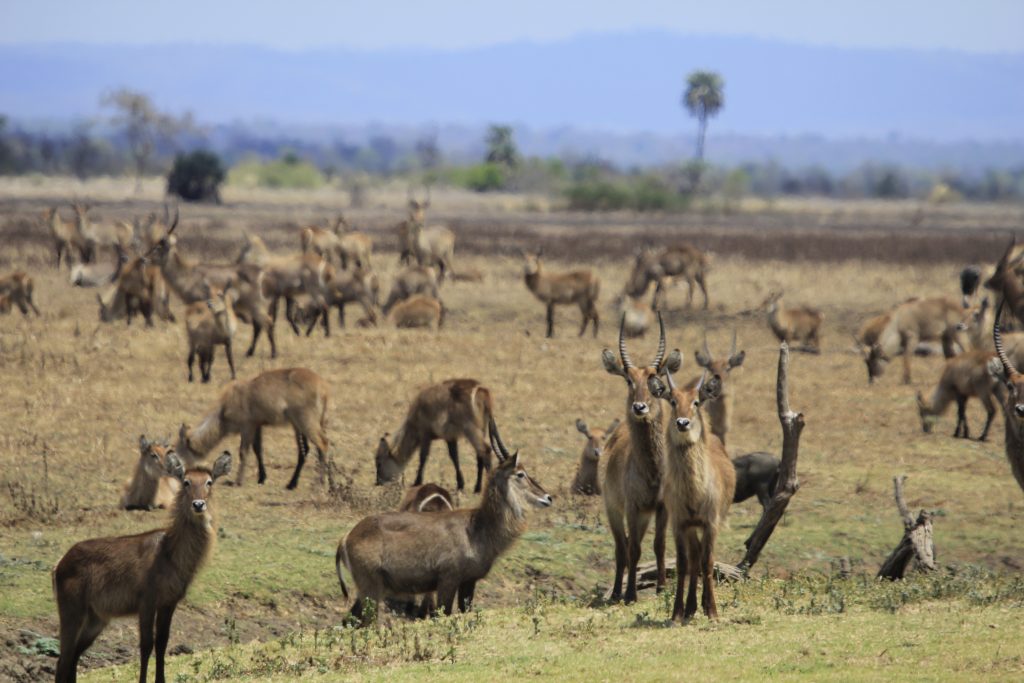
SB:
What are the paradoxes that emerge when you study resource partitioning among diverse large-herbivore assemblages in Gorongosa?
AP:
The biggest paradox is that the world is green. Plants are abundant, yet only a small fraction of plant matter gets eaten. In many ecosystems, only about 10% of the annual net primary productivity (a measure of how much plant matter is produced) gets eaten. The rest, which is uneaten, eventually falls to the ground and decays or might be burned in a fire. In some systems, such as the vast Serengeti grasslands, the amount of plant material eaten by wildebeest and zebras and the like might exceed 50%; stil, lots of grass goes to waste. Why? Is it not edible? Or do predators limit herbivore populations and prevent the herbivores from eating everything? This is one of the most puzzling and interesting questions in ecology.
In terms of edibility, some scientists point out that plants have all sorts of defenses against herbivores—spines, sticky sap, potent chemicals, indigestible woody fiber, etc. So plants might be fending off herbivores. At the same time, herbivores have evolved all sorts of ways to overcome these defenses—beneficial detoxifying gut bacteria, strong teeth to chew up fiber, enzymes to break down food, brains to figure out what to eat, etc. We humans have developed additional mechanisms—we chop and cook our food. The coevolution of herbivores and plants is ancient; plants have elaborate ways to avoid getting eaten (or to be eaten on their terms, the way a strawberry plant advertises its ripe fruit), and herbivores have elaborate ways to deal with plants and make them into food.
The coevolution of plants and herbivores is so extensive that plants and herbivores might even ‘need the struggle’ somewhat. On the plant side, certain short-growing grasses actually do better if they are grazed (or mowed!) into a lawn. For instance, cows are adapted to eating low-quality grass, but they grow faster when fed corn–the problem is that too much corn gives animals health problems. Monarch butterfly caterpillars feed on milkweed, a plant loaded with toxic chemicals that we as humans can only eat after extensive cooking; these caterpillars are so adapted to feed on milkweed that they don’t do well on anything else!
The implications of coevolution for humans are huge—humans are remarkably good at achieving rich, nutritious diets. People (like animals) don’t need to read nutrition labels to get a balance of proteins, fats, and carbs—our brain steers us towards a healthy mix most of the time. And these biological adaptations have even been built into our food culture, in both traditional food pairings (potatoes & meat, rice & beans, bread & cheese) and in certain dieting approaches (intuitive eating). Broadly speaking, humans know what is good for them.
At the same time, the coevolutionary history of humans and plants suggests that we might do better with a bit more struggle. We know we should be getting more fiber and eating more veggies. But our domesticated foods are often milder and less pungent tasting than their wild counterparts, which were the foods our ancestors ate. I would speculate that we’ll discover that humans should add some of that pungency back into food for the best health—the bitter apple, the spicy mustard green, the puckeringly astringent tea all may be giving our bodies and the microbes within them a ‘useful challenge,’ in the same way that exercise benefits our bodies.
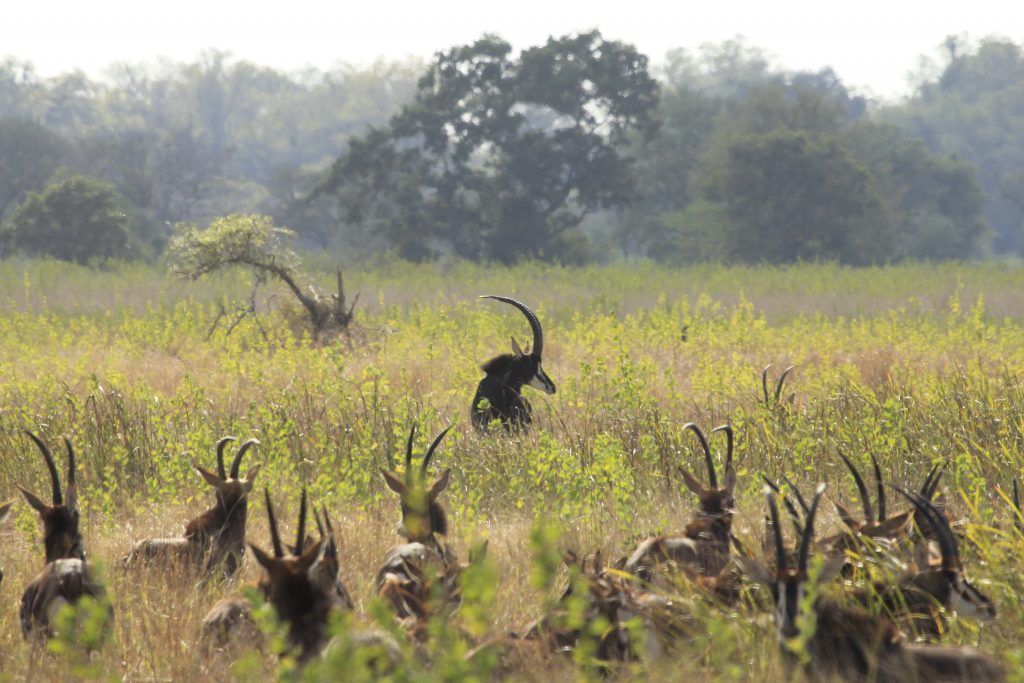
SB:
What are the ramifications of studying grasslands on agricultural practices?
AP:
We have a lot to learn from studying grasslands. To start, the grass family is found worldwide, and grasses form the major part of the vegetation of large areas of the world, from the arctic tundra through steppes and prairies to tropical savannas. Grasses are extremely important economically—corn, wheat, rice, and most other grain crops are grasses; grasses are important forage for livestock. Bamboo is a grass, and it finds all sorts of fascinating uses. Grasses which use the C4 photosynthetic pathway are able to grow extremely quickly in hot and dry conditions, which will make them very important for combating climate change and food insecurity. So studying grasslands is of global importance, despite the fact that they don’t get the same attention that forests get.
My own research relates to agriculture in a few different ways. For instance, we did a study on elephants in Gorongosa; some elephants were crossing out of the national park and raiding the fields of farmers. This sort of human-wildlife conflict is a major challenge and solving it is not easy. Using DNA metabarcoding and nutritional analysis, we showed that the elephants were after energy-rich foods and not protein; this informs which crops are most likely to be raided and which crops will be ignored by elephants. Another useful finding in Gorongosa National Park was that large herbivores were controlling the spread of a noxious invasive weed called Mimosa pigra. Some public agencies spend millions of dollars trying to control this plant; Gorongosa’s diverse herbivore community keeps Mimosa in check. This specific finding relates to the broader implications of my research, which shows that different herbivore species complement each other. Diverse herbivore communities—whether wild or domestic—will control weeds, disperse seeds, spread fertilizer, and shape the habitat for one another and for other species; it is also likely that this makes landscapes more adaptable in the face of climate change and more resistant to disease outbreaks.
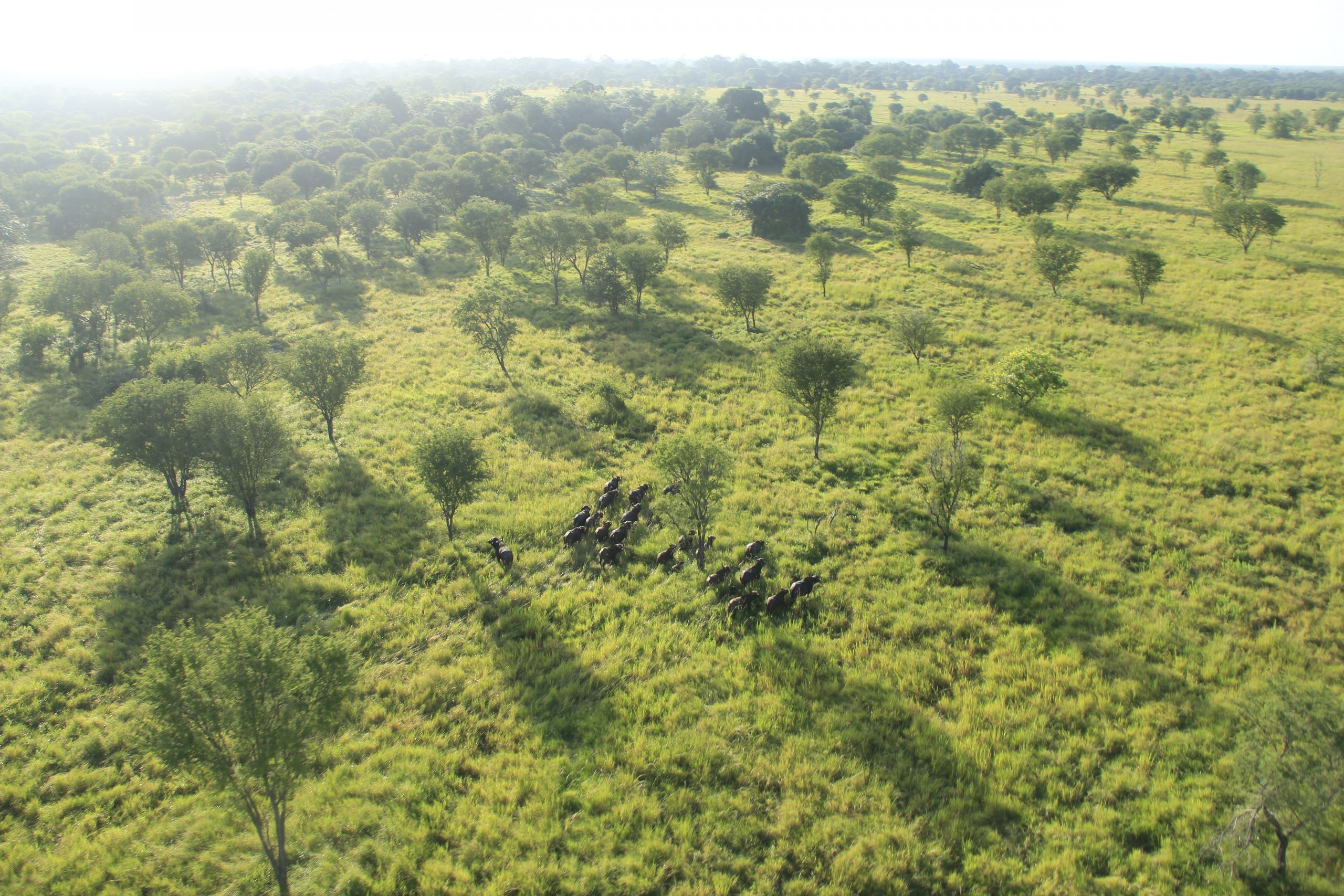
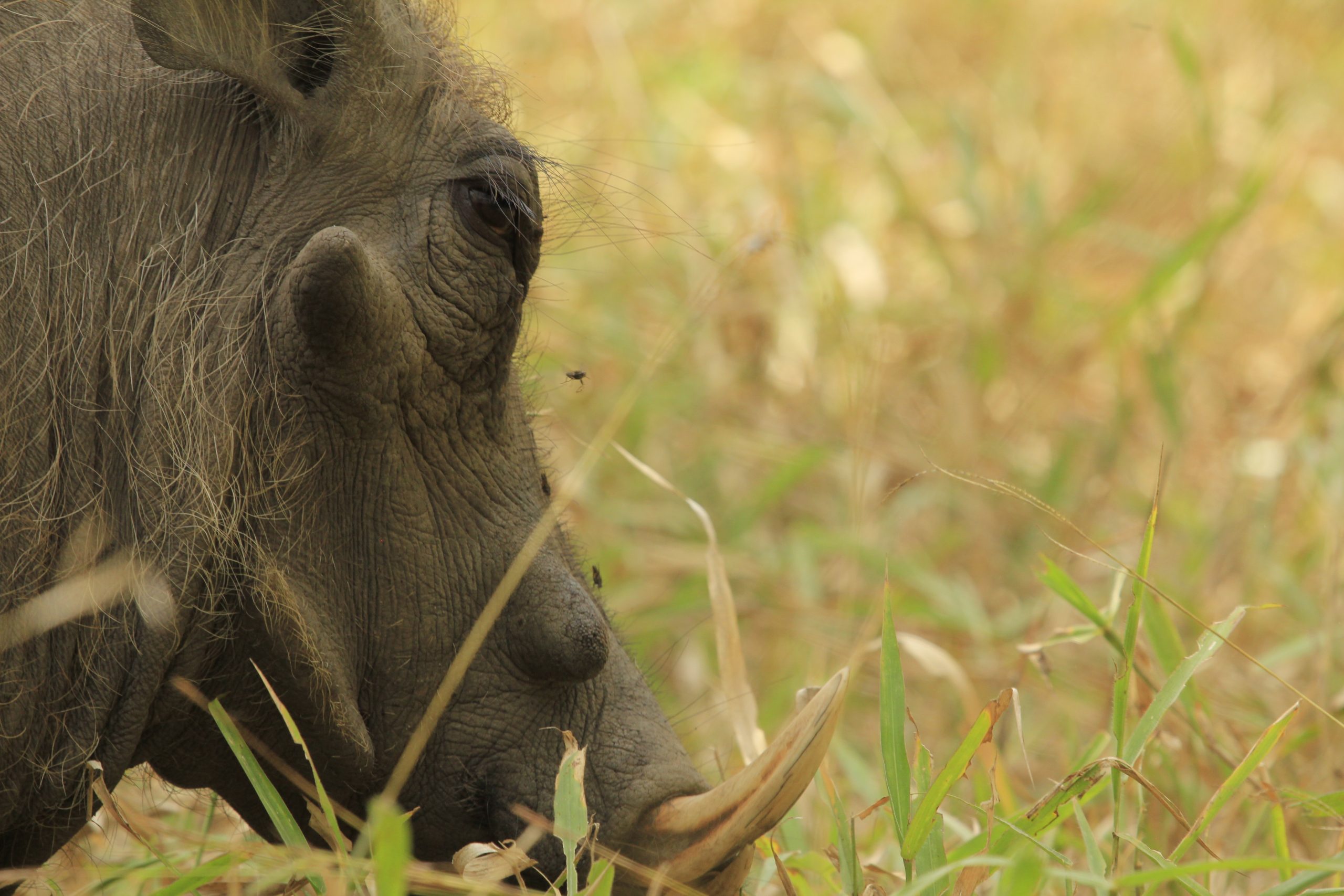
SB:
What is the significance of DNA Metabarcoding in your field-work?
AP:
DNA metabarcoding is a relatively new technique that is used to ‘barcode’ or identify many species at once using their DNA. Regular DNA barcoding is a powerful tool that finds all sorts of uses— it can be used to discover new species of animals or test if the seafood you buy is mislabeled. By barcoding many species at once, researchers can unlock worlds that were previously invisible or difficult to study. For instance, a friend of mine looked for ancient elephant DNA buried in the lakeside mud in the Alps, as a way to see the path that Hannibal’s army took when invading Italy (while he found no elephant DNA, he did find evidence of ancient Roman cheesemaking).. One of the biggest uses of this technique these days is characterizing the complex flora of bacteria and yeast and other things that live in and on our bodies; what we call the microbiome. It turns out that most of the little organisms that live around us cannot be cultured very easily and thus were difficult to study; DNA metabarcoding is changing that, and we are learning more and more about how important microbes are to human health.
In my research, we use DNA metabarcoding to describe diets in great detail, using only a small fecal sample. We extract and amplify plant DNA and match these DNA sequences to a library of known plant species—in this way, we can make a list of the plants that an animal has eaten and estimate their proportional contribution to diet. This new method has led to a number of intriguing findings— some collaborators have found that two species of zebra living in the same region of Kenya both eat mostly grasses, but they actually eat different species of grasses. Such a finding would not have been possible until recently, since it is difficult to actually see and identify the plants that a wild animal is eating.
My work (together with collaborators) builds on these diet studies to ask how the plant species that one herbivore eats differ from the plant species another herbivore eats. This has led to a number of interesting findings3. One example is that we find that animals with a shorter shoulder height eat more of plant species that are short. For instance, zebras (being somewhat tall) eat a lot of tall, bushy grass species, while the short-statured warthog eats many creeping grasses, of the varieties that you might find in lawns and sports fields.
- 3. The height-stratification hypothesis essentially suggests that taller animals would forage at higher levels than their shorter counterparts. This would facilitate dietary niche partitioning–that is, it would differentiate the diets, which could minimize competition for scarce resources. As an example, a goat and a camel might feed on the same Acacia tree–the goat would nibble small branches near the base, while the camel would chomp foliage higher up on the tree. A giraffe, in turn, would have exclusive access to the highest branches, which only it could reach with its long neck.
SB:
How is the ecological/scientific breakdown of ‘nutrition’ similar or different from our general understanding of it?
AP:
Animals need to worry about getting enough energy and calories to survive lean periods and to reproduce. The foods that are most ‘nutritious’ in this context are those that are highly digestible and rich in calories and protein, since these will help animals put on extra body fat to buffer against malnutrition. So, in a sense, what is ‘nutritious’ for animals aligns pretty closely with what we would call ‘fattening’ or ‘rich’ or ‘caloric.’
The problem of getting enough nutrition is particularly tricky for large herbivores—harvesting leaves and tender twigs is hard work, and digesting them and getting sufficient energy and nutrients is not easy. Imagine if you had to put on weight while eating nothing but salad. It would be difficult. But this is exactly what a cow does when it turns grass into meat and milk.
Humans have been hardwired by natural selection to seek out energy-rich food; with agriculture, we’ve created a world where calorie-rich food is easier than ever to obtain.
SB:
Why is Gorongosa a relevant site to conduct your research? Also what is the relationship like between the locals and the national park?
AP:
In terms of my research, Gorongosa National Park in Mozambique is an excellent place to work. The park has one of the most impressive large herbivore assemblages in the world; many species that are rare in the rest of Africa (such as the majestic Sable antelope) are thriving in Gorongosa. In all, Gorongosa has more than 20 species of hoofed animals, which is more than you would find in the entire United States.
Gorongosa is also a very exciting place to work because it defines a new paradigm for conservation and human development. In terms of science, the park is training a new generation of Mozambican scientists, some of whom are my collaborators. The park has actually created the first Master’s program in conservation biology in the country. While Gorongosa is an amazing place to be a foreign tourist on safari, the park is also visited by thousands of local people. Recently, the park has successfully experimented with using shade-grown coffee as a way to provide employment and regenerate rainforests simultaneously. In all, places like Gorongosa leave me hopeful that protected areas—which are essential to conserving biodiversity—can also serve as engines of inclusive economic development in rural areas.
SB:
What should we understand and do better for a better ecological and dietary future?
AP:
It is worth remembering that all organisms—including humans—interact and evolve with each other. These interactions can be positive or negative or neutral; we are all shaping and being shaped by the larger ecological systems of which we are just a part.
In terms of what we can do for our health, we would be better off eating a diverse diet with more vegetables and more leafy greens in particular. For the sake of the climate, we’d do well to reduce our consumption of ruminant meat (esp. beef) and dairy, since these are associated with high emissions of the greenhouse gas methane. At the same time, animal agriculture can be made compatible with local biodiversity conservation, and there may be strategies to mitigate this climate impact. Restoring native large animal assemblages delivers a whole slew of benefits, from tourism to nutrient cycling to weed control. Protected areas (such as national parks) are probably the single best strategy for conserving the world’s biodiversity and maintaining a healthy environment for humans. But it is also important to remember that you don’t need to go to a national park to enjoy nature – ecology is everywhere, even in your stomach.